CRISPR/Cas9 is a gene editing tool that has revolutionized biomedical research and led to the first FDA-approved CRISPR-based gene therapy. However, until now, the precise mechanism of exactly how this tool works and avoids creating detrimental off-target effects was not well understood. Now, using state-of-the-art technology, researchers from Children’s Hospital of Philadelphia (CHOP) have identified several specific steps needed for CRISPR to become active and perform its gene editing function. These preclinical findings could lead to improved designs for CRISPR-based gene editing. The findings were published today in the journal Cell Chemical Biology.
Gene editing allows genetic material to be added, removed or altered in some other way on a person’s genome. In healthcare, it has enormous potential to “correct” mutated genes that form the basis of many rare and complex diseases. However, for gene editing to be effective, it needs to be precise and only edit at an intended target.
While clinical trials have explored the direct delivery of Cas9 to patients through lipid nanoparticles for gene editing since 2020, broad applicability is challenging. Cas9 has the potential to perform unintended genome edits outside the target gene that could be harmful and could even turn cells cancerous. Therefore, cells taken from a patient are used to perform gene editing outside the body before delivering them back into the patient. This is an expensive and time-consuming process, driven by an incomplete understanding of gene editing mechanisms.
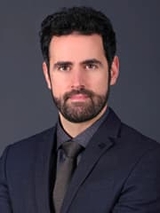
“We always had a suspicion that what we could see from existing structures from the gene editing process was not telling us the whole story,” said senior study author Nikolaos G. Sgourakis, PhD, Associate Professor in the Center for Computational and Genomic Medicine at CHOP and Associate Professor in the Department of Biochemistry and Biophysics at the University of Pennsylvania Perelman School of Medicine.
In this study, researchers used a technique called nuclear magnetic resonance spectroscopy to visualize atoms and proteins as well as their dynamics to observe how they move between different states. This method allowed the researchers to observe CRISPR as it transitions from an inactive to an active state and the steps required to make this happen, which was not possible without this state-of-the-art equipment.
Operating like a proofreader within an assembly line, an intermediate “surveillance” structure serves as a gatekeeper to regulate the enzyme’s DNA cutting activity. This step is key to discriminate between off- and on-target DNA sequences by the Cas9 protein that match its programmable guide RNA, a short sequence that directs DNA editing to precise locations in the genome. There are many engineered variants of Cas9, and higher fidelity variants favor the recognition of on-target DNA and help stabilize the “surveillance” complex while this happens, thereby reducing off-target effects and making sure therapy is delivered in a more precise manor.
Understanding these underlying mechanisms of how CRISPR and Cas9 operate could lead to more effective forms of gene editing technology, including the potential to deliver the therapy directly into the body, and could even improve other therapies such as CAR-T cell therapy.
“It’s much easier to deliver the pre-programmed Cas9 complex in the form of a lipid nanoparticle to directly edit target cells in the patient when we can be sure that it’s going to do its job correctly,” Sgourakis said. “For example, precise gene editing using CRISPR technology could be used to directly modify T cells in patients, and that would allow us to simplify the adaptation of CAR-Ts and other cellular therapies.”
Nuclear magnetic resonance spectroscopy for this study was performed at the Institute of Structural Biology at the University of Pennsylvania, using shared instrumentation from the Perelman School of Medicine at the University of Pennsylvania and CHOP.
This study was supported by the National Institute of Allergy and Infectious Diseases of the National Institutes of Health grant NIH R01AI143997, the NexTGen team through the Cancer Grand Challenges partnership, Cancer Research UK grant CGCAT F-2021/100002, the National Cancer Institute grant CA 278687-01, and the Mark Foundation for Cancer Research. Further funding was provided by NIH NIGMS grants R35GM125034 and 3R35GM125034-05S, NIH grant GM136859 and the Claudia Adams Barr Program for Innovative Cancer Research.
De Paula et al, “Dynamic sampling of a surveillance state enables DNA proofreading by Cas9.” Cell Chem Biol. Online October 28, 2024. DOI: 10.1016/j.chembiol.2024.10.001
Featured in this article
Experts
Research
CRISPR/Cas9 is a gene editing tool that has revolutionized biomedical research and led to the first FDA-approved CRISPR-based gene therapy. However, until now, the precise mechanism of exactly how this tool works and avoids creating detrimental off-target effects was not well understood. Now, using state-of-the-art technology, researchers from Children’s Hospital of Philadelphia (CHOP) have identified several specific steps needed for CRISPR to become active and perform its gene editing function. These preclinical findings could lead to improved designs for CRISPR-based gene editing. The findings were published today in the journal Cell Chemical Biology.
Gene editing allows genetic material to be added, removed or altered in some other way on a person’s genome. In healthcare, it has enormous potential to “correct” mutated genes that form the basis of many rare and complex diseases. However, for gene editing to be effective, it needs to be precise and only edit at an intended target.
While clinical trials have explored the direct delivery of Cas9 to patients through lipid nanoparticles for gene editing since 2020, broad applicability is challenging. Cas9 has the potential to perform unintended genome edits outside the target gene that could be harmful and could even turn cells cancerous. Therefore, cells taken from a patient are used to perform gene editing outside the body before delivering them back into the patient. This is an expensive and time-consuming process, driven by an incomplete understanding of gene editing mechanisms.
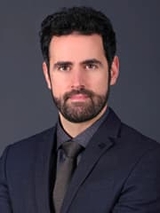
“We always had a suspicion that what we could see from existing structures from the gene editing process was not telling us the whole story,” said senior study author Nikolaos G. Sgourakis, PhD, Associate Professor in the Center for Computational and Genomic Medicine at CHOP and Associate Professor in the Department of Biochemistry and Biophysics at the University of Pennsylvania Perelman School of Medicine.
In this study, researchers used a technique called nuclear magnetic resonance spectroscopy to visualize atoms and proteins as well as their dynamics to observe how they move between different states. This method allowed the researchers to observe CRISPR as it transitions from an inactive to an active state and the steps required to make this happen, which was not possible without this state-of-the-art equipment.
Operating like a proofreader within an assembly line, an intermediate “surveillance” structure serves as a gatekeeper to regulate the enzyme’s DNA cutting activity. This step is key to discriminate between off- and on-target DNA sequences by the Cas9 protein that match its programmable guide RNA, a short sequence that directs DNA editing to precise locations in the genome. There are many engineered variants of Cas9, and higher fidelity variants favor the recognition of on-target DNA and help stabilize the “surveillance” complex while this happens, thereby reducing off-target effects and making sure therapy is delivered in a more precise manor.
Understanding these underlying mechanisms of how CRISPR and Cas9 operate could lead to more effective forms of gene editing technology, including the potential to deliver the therapy directly into the body, and could even improve other therapies such as CAR-T cell therapy.
“It’s much easier to deliver the pre-programmed Cas9 complex in the form of a lipid nanoparticle to directly edit target cells in the patient when we can be sure that it’s going to do its job correctly,” Sgourakis said. “For example, precise gene editing using CRISPR technology could be used to directly modify T cells in patients, and that would allow us to simplify the adaptation of CAR-Ts and other cellular therapies.”
Nuclear magnetic resonance spectroscopy for this study was performed at the Institute of Structural Biology at the University of Pennsylvania, using shared instrumentation from the Perelman School of Medicine at the University of Pennsylvania and CHOP.
This study was supported by the National Institute of Allergy and Infectious Diseases of the National Institutes of Health grant NIH R01AI143997, the NexTGen team through the Cancer Grand Challenges partnership, Cancer Research UK grant CGCAT F-2021/100002, the National Cancer Institute grant CA 278687-01, and the Mark Foundation for Cancer Research. Further funding was provided by NIH NIGMS grants R35GM125034 and 3R35GM125034-05S, NIH grant GM136859 and the Claudia Adams Barr Program for Innovative Cancer Research.
De Paula et al, “Dynamic sampling of a surveillance state enables DNA proofreading by Cas9.” Cell Chem Biol. Online October 28, 2024. DOI: 10.1016/j.chembiol.2024.10.001
Recommended reading
Researchers Discover Deep Structural Biology Connections that Help Improve CAR Therapy
Identifying “backbones” that link complexes can help maximize CAR therapy across different variants and tumor types.
Contact us
Ben Leach